Translate this page into:
Decreased SIRT1 protein may promote HMGB1 translocation in the keratinocytes of patients with cutaneous lupus erythematosus
Corresponding author: Dr. Yaping Li, Department of Dermatology, Hunan Key Laboratory of Medical Epigenomics, The Second Xiangya Hospital, Central South University, No. 139, Renmin Middle Road, Furong District, Changsha, Hunan, China. lyplisa@csu.edu.cn
-
Received: ,
Accepted: ,
How to cite this article: Zhou X, Qiu Y, Mu K, Li Y. Decreased SIRT1 protein may promote HMGB1 translocation in the keratinocytes of patients with cutaneous lupus erythematosus. Indian J Dermatol Venereol Leprol. 2024;90:425-32. doi: 10.25259/IJDVL_814_2022
Abstract
Background
Ultraviolet radiation causes DNA damage in keratinocytes, aggravating cutaneous lupus erythematosus (CLE). High mobility group box 1 (HMGB1) participates in nucleotide excision and may transfer from the nucleus to the cytoplasm in immune active cells and the translocation of HMGB1 may result in DNA repair defects. HMGB1 was observed to transfer from the nucleus to the cytoplasm in the keratinocytes of CLE patients. As a class III histone deacetylases (HDACs), sirtuin-1 (SIRT1) can induce HMGB1 deacetylation. Epigenetic modification of HMGB1 may lead to HMGB1 translocation.
Aims
We aimed to evaluate the expressions of SIRT1 and HMGB1 in the epidermis of CLE patients and whether decreased SIRT1 leads to HMGB1 translocation through HMGB1 acetylation in keratinocytes.
Methods
We measured the messenger RNA (mRNA) and protein expressions of SIRT1 and HMGB1 in CLE patients using real-time reverse transcription polymerase chain reaction (RT-qPCR) and western blotting. Keratinocytes were treated with SIRT1 activator resveratrol (Res) and irradiated with ultraviolet B (UVB). We detected the localization expression of HMGB1 by immunofluorescence. The apoptosis level and the cell cycle proportions were measured by flow cytometry. The acetyl-HMGB1 level was detected by immunoprecipitation.
Results
Compared to healthy controls, the mRNA and protein expressions of SIRT1 in the epidermis of CLE patients were significantly decreased and there was translocation of HMGB1 from the nucleus to the cytoplasm. In keratinocytes, UVB irradiation led to HMGB1 translocation from the nucleus to the cytoplasm. Res treatment inhibited HMGB1 translocation, attenuated the cell apoptosis induced by UVB and decreased the acetyl-HMGB1 level.
Limitations
We only treated keratinocytes with the SIRT1 activator but did not perform the relevant experiments in keratinocytes with SIRT1 knockdown or overexpression. In addition, the lysine residue site of action of SIRT1 deacetylation of HMGB1 is unclear. The specific mechanism of action of SIRT1 deacetylation of HMGB1 needs to be further investigated.
Conclusion
SIRT1 may inhibit HMGB1 translocation by HMGB1 deacetylation which inhibited the apoptosis of keratinocytes induced by UVB. Decreased SIRT1 may promote HMGB1 translocation in the keratinocytes of patients with CLE.
Keywords
Cutaneous lupus erythematosus
keratinocytes
HMGB1
SIRT1
Plain Language Summary
Translocation of high mobility group box 1 (HMGB1) from the nucleus to the cytoplasm may related to autoimmunity. Epigenetic modification of HMGB1 may lead to HMGB1 translocation. This study was conducted to evaluate the expressions of the histone deacetylase sirtuin-1 (SIRT1) and HMGB1 in the epidermis of cutaneous lupus erythematosus (CLE) patients and to understand whether decreased SIRT1 leads to HMGB1 translocation through HMGB1 acetylation in keratinocytes. The expressions of SIRT1 and HMGB1 were measured in the epidermis of CLE patients. The localization expression of HMGB1 and the acetyl-HMGB1 level were detected in keratinocytes treated with SIRT1 activator resveratrol (Res) and UVB irradiation. The results suggested that decreased SIRT1 may promote HMGB1 translocation in CLE keratinocytes. And SIRT1 may inhibit HMGB1 translocation by HMGB1 deacetylation in keratinocytes.
Introduction
Lupus erythematosus (LE) is an autoimmune disease including cutaneous LE (CLE) and systemic LE (SLE). Skin lesions are the main clinical manifestation of CLE and ultraviolet B (UVB) exposure can trigger and aggravate skin lesions in CLE. UV-induced DNA damage activates DNA repair, whereas defects in DNA damage repair may lead to apoptosis, tissue inflammation and autoimmunity.1
Increased keratinocyte apoptosis strongly correlated with poor prognosis of CLE.2,3 In the tissues and circulation of SLE patients, there is an accumulation of apoptotic cells presenting autoantigens and releasing high mobility group box 1 (HMGB1).4,5
As a member of the high mobility group protein family, HMGB1 is an important non-histone nuclear protein6 that is widely present in the nucleus. Nuclear HMGB1 binds to the DNA and facilitates transcription, replication and repair.7 When cells are damaged or activated, HMGB1 is released from the nucleus into the cytoplasm and subsequently into the extracellular compartment, thereby activating the immune system and promoting inflammatory responses.8 The nuclear localization and affinity of HMGB1 to DNA are mainly regulated by post-translational modifications of HMGB1, such as acetylation and phosphorylation.9
The sirtuin (SIRT) family is a homologous deacetylase family of silencing information regulatory factor 2 (Sir2),10 of which SIRT1 is the first recognised and most extensively studied protein. SIRT1 belongs to class III histone deacetylases (HDACs).11 In the mouse mononuclear macrophage cell line RAW264.7, SIRT1 was shown to bind with HMGB1 to form a complex and induce HMGB1 deacetylation by binding to its lysine residue (Lys), thereby inhibiting the translocation and extracellular release of HMGB1.12
We evaluated the involvement of HMGB1 in the development of skin damages in CLE patients after UVB exposure and whether decreased SIRT1 leads to HMGB1 translocation through HMGB1 acetylation in keratinocytes.
Methods
Patients and controls
The CLE patients (n = 9, mean age = 35.1 ± 14.2 years) were enrolled from the outpatient and inpatient departments of the Second Xiangya Hospital, Central South University, Hunan, China. We also enrolled healthy controls (n = 9, mean age = 32.4 ± 10.7 years) whose age and gender matched with the experimental group. CLE patients mainly include discoid lupus erythematosus (DLE) patients and subacute cutaneous lupus erythematosus (SCLE) patients. Typical lesions of DLE are flat or slightly raised at the edges with a slightly depressed central discoid erythema. The two main types of SCLE are annular erythema type and papular scaly type. CLE patients generally have no or mild systemic involvement and are examined for dermatopathology and immunopathology in addition to clinical lesions and immunological indicators. Skin lesions on the exposed areas of patients and controls were selected. The skin lesions of healthy controls were normal skin tissue from patients undergoing nevus excision. The human sample research was evaluated and endorsed by the human ethics committee of our hospital (research project: study on the pathogenesis of autoimmune diseases and inflammatory skin diseases [SADISD]; scheme number: SADISD 20190220).
Epidermis separation
The skin tissues from patients and controls were collected by skin biopsy while the skin tissues for isolating normal human epidermal keratinocytes (NHEKs) were collected by circumcision from healthy individuals who had previously undergone circumcision for non-study-related reasons. The collected skin tissues were cut to the size of 0.5 cm × 0.5 cm and preserved in 2 mg/mL of dispase II enzyme solution (MERCK, NJ, USA) containing 5% FBS (Gibco, Grand Island, NY, USA) and 1% penicillin/streptomycin at 4°C for 12 h. The epidermis was separated from the dermis and subcutaneous tissue using scissors and forceps and the epidermis was then preserved.
Cell isolation and culture
The separated epidermis was cut into pieces and digested by 0.25% Trypsin-EDTA (Gibco) to obtain NHEKs which were then maintained in K-SFM (Gibco) with a growth supplement and 1% penicillin/streptomycin. HaCaT cells were maintained in DMEM (Gibco) with 10% FBS and 1% penicillin/streptomycin. The cells were incubated at 37°C in 5% CO2 and 95% humidified air.
UVB irradiation
In an ultra-clean sterile cell bench, the cells were irradiated with UVB when the cell density reached approximately 70% (for immunocytofluorescence experiment, the cell density was 40%). The height of the UV lamp (Philips, Amsterdam, NLD) was adjusted until the UV irradiation meter (Beijing Shida Photoelectric Technology Ltd., Beijing, China) showed 200 µW/cm2 and the corresponding irradiation times (0 s, 150 s) were determined according to the different irradiation doses (0 mJ/cm2, 30 mJ/cm2). We aspirated the spent medium from the culture dishes, added a small amount of Dulbecco’s phosphate-buffered saline (DPBS) to prevent the cells from drying out during irradiation and removed the lid for UVB irradiation. After UVB irradiation, we aspirated the DPBS, added the appropriate amount of medium with or without resveratrol (Res) and incubated the cells in an incubator at 37°C for 24 h; we then collected the cells for subsequent experiments.
RNA isolation and RT-qPCR
We extracted the total RNA from the epidermal tissue and keratinocytes using TRIzol reagent, followed by treatment with PrimeScript RT reagent kit and gDNA Eraser (TaKaRa, Shiga, Japan) to synthesise the cDNA. The cDNA was used as an amplification template to amplify the target genes and GAPDH using RT-qPCR and an SYBR Premix Ex Taq Kit (TaKaRa). The transcription levels of SIRT1, HMGB1 and GAPDH were detected using a TB Green Premix Ex Taq (TaKaRa). The primers used for RT-qPCR were 5′-AAGGCTGGGGCTCATTTG-3′ and 5′-TCTTGAGGCTGTTGTCATACTTC-3′ (for GAPDH), 5′-GCCTCACATGCAAGCTCTAGTGAC-3′ and 5′-TTCGAGGATCTGTGCCAATCATAA-3′ (for SIRT1) and 5′-ATGGACAAAACCCCTCTTC-3′ and 5′-TCTAAATTGCCTCCCACTTC-3′ (for HMGB1). The 2−ΔΔCt method (ΔCt = Ctaim proteins − CtGAPDH; ΔΔCt = ΔCtexperimental group − Δ Ctcontrol group) was used to determine the cDNA value; these values were standardised to GAPDH.
Western blot
The total proteins from the epidermal tissue and keratinocytes were isolated and quantified. The primary antibodies used included anti-SIRT1 (1:1000; Cell Signaling Technology, Danvers, MA, USA), anti-HMGB1 (1:10000; Abcam, Cambridge, MA, USA), acetylated lysine (1:1000; Cell Signaling Technology), β-actin (1:2000; Proteintech, Chicago, IL, USA), GAPDH (1:5000; Proteintech) and lamin B1 (1:2000; Proteintech). The secondary antibody used was HRP-conjugated Goat Anti-Rabbit IgG (H+L) (1:5000; Proteintech). We exposed the western blots to X-ray and quantified the band densities using Image-Pro Plus software. Densitometry was used to normalise the protein levels to those of β-actin, GAPDH or lamin B1.
Apoptosis detection
Cell apoptosis was detected using the Annexin V FITC Apoptosis Detection kit Ⅱ (BD Biosciences, San Diego, CA, USA) according to the instructions. The experiment was carried out at room temperature and the cells were kept from being exposed to light. The cell precipitate was collected and 100 µl of diluted 1× binding buffer was added to each tube to resuspend the cells. Then the cells were incubated with Annexin V-FITC and PI and were resuspended with 400 µl of diluted 1× binding buffer. Finally, we used flow cytometry (FACSCanto-Ⅱ; BD Biosciences) to detect cell apoptosis.
Cell cycle detection
The trypsinised cells were fixed with 70% ethanol at −20°C for more than 4 hours. Cell cycle was detected using the PI/RNase Staining Buffer (BD Biosciences) in accordance with the instructions. We used flow cytometry to detect the cell cycle.
Immunohistofluorescence
The nuclear-cytoplasmic localization expression of HMGB1 in the epidermis lesions of CLE patients and healthy controls was detected using immunohistofluorescence with the Opal Automation Multiplex IHC Detection Kits (Akoya Biosciences, Menlo Park, CA, USA). Paraffin slices were baked in a hybrid furnace at 70°C and dewaxed by soaking in turpentine and gradient concentration of alcohol. The antigen was retrieved using high-pressure antigen retrieval. The antigen was incubated with the primary antibody (anti-HMGB1; 1:6000; Abcam) and the secondary antibody (Opal Polymer HRP MS + Rb), OPAL 620 reagent (1:200) followed by DAPI. Multispectral fluorescence microscopy was used to observe and photograph the experimental results. For each sample, three typical fields of view were selected and analysed using Inform software to calculate the cytoplasmic positivity rate. The average results of the three fields of view were used for statistical analysis.
Immunocytofluorescence
The nuclear-cytoplasmic localization expression of HMGB1 in the keratinocytes was detected using immunocytofluorescence. The cells were fixed in 4% paraformaldehyde. Then, we used 0.5% Triton X-100 to permeate the membrane. The cells were incubated with the primary antibody (anti-HMGB1; 1:250; Abcam) and the fluorescent secondary antibodies, Alexa flour 488 (1:500; Servicebio, Wuhan, China) followed by DAPI. The multispectral fluorescence microscopy was used to observe and photograph the experimental results. The statistical analysis was consistent with that used for immunocytofluorescence.
Immunoprecipitation
Briefly, the protein isolated using immunoprecipitation (IP) lysis buffer (Beyotime, Shanghai, China) was pre-purified with Protein G Agarose (Beyotime). The protein was incubated with anti-HMGB1 antibody (1:100; Abcam) or IgG (1:100; Proteintech). Protein G Agarose was added to capture the immunoprecipitated protein complex. The partners of HMGB1 were identified by immunoblots.
Statistical analysis
We used the GraphPad Prism 8 software for statistical analysis. The results are presented as mean ± standard deviation. Unpaired Student’s t-test was used when data were normally distributed and displayed as homogeneous variances. Otherwise, the Mann–Whitney U test was used. P values <0.05 were considered significant.
Results
SIRT1 and HMGB1 levels in the epidermis of CLE patients
We analysed the epidermal SIRT1 and HMGB1 levels by RT-qPCR and western blotting. In contrast to healthy controls, the SIRT1 mRNA and protein expression were significantly decreased in CLE patients (1.17 ± 0.72 vs. 0.57 ± 0.16, P = 0.025, n = 9 for mRNA; 0.76 ± 0.26 vs. 0.36 ± 0.10, P = 0.006, n = 6 for proteins; Figures 1a, 1c, 1d). No significant difference was found in the HMGB1 mRNA and protein expression levels between the groups (1.35 ± 1.12 vs. 1.61 ± 0.70, P = 0.572, n = 9 for mRNA; 0.90 ± 0.19 vs. 0.70 ± 0.18, P = 0.810, n = 6 for proteins; Figures 1b, 1c, 1e).
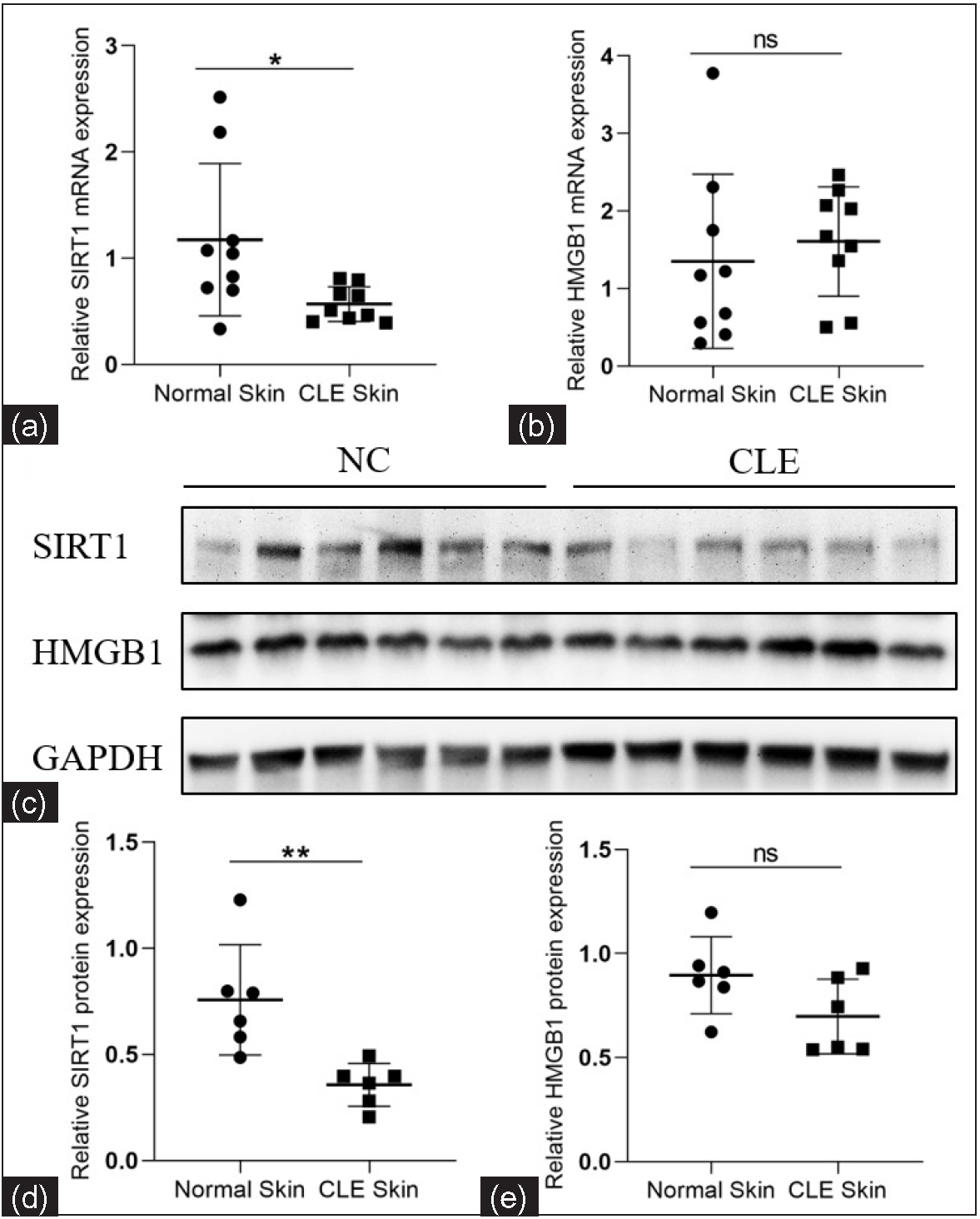
- Relative mRNA and protein expression levels of SIRT1 and HMGB1 in the epidermis of CLE patients and healthy controls. (a, b) Comparison of the SIRT1 and HMGB1 mRNA expression levels between CLE patients (n = 9) and healthy controls (n = 9). (c–e) Comparison of the SIRT1 and HMGB1 protein expression levels between CLE patients (n = 6) and healthy controls (n = 6) (*P < 0.05, **P < 0.01, ns P > 0.05).
Nuclear-cytoplasmic localization expression of HMGB1 in the epidermis of CLE patients
The nuclear-cytoplasmic localization expression of HMGB1 in the epidermis was detected using immunohistofluorescence. Compared to healthy controls, the cytoplasmic positivity rate (%) for HMGB1 was significantly increased in CLE patients (1.89 ± 2.57 vs. 36.90 ± 16.57, P = 0.002, n = 5; Figures 2).
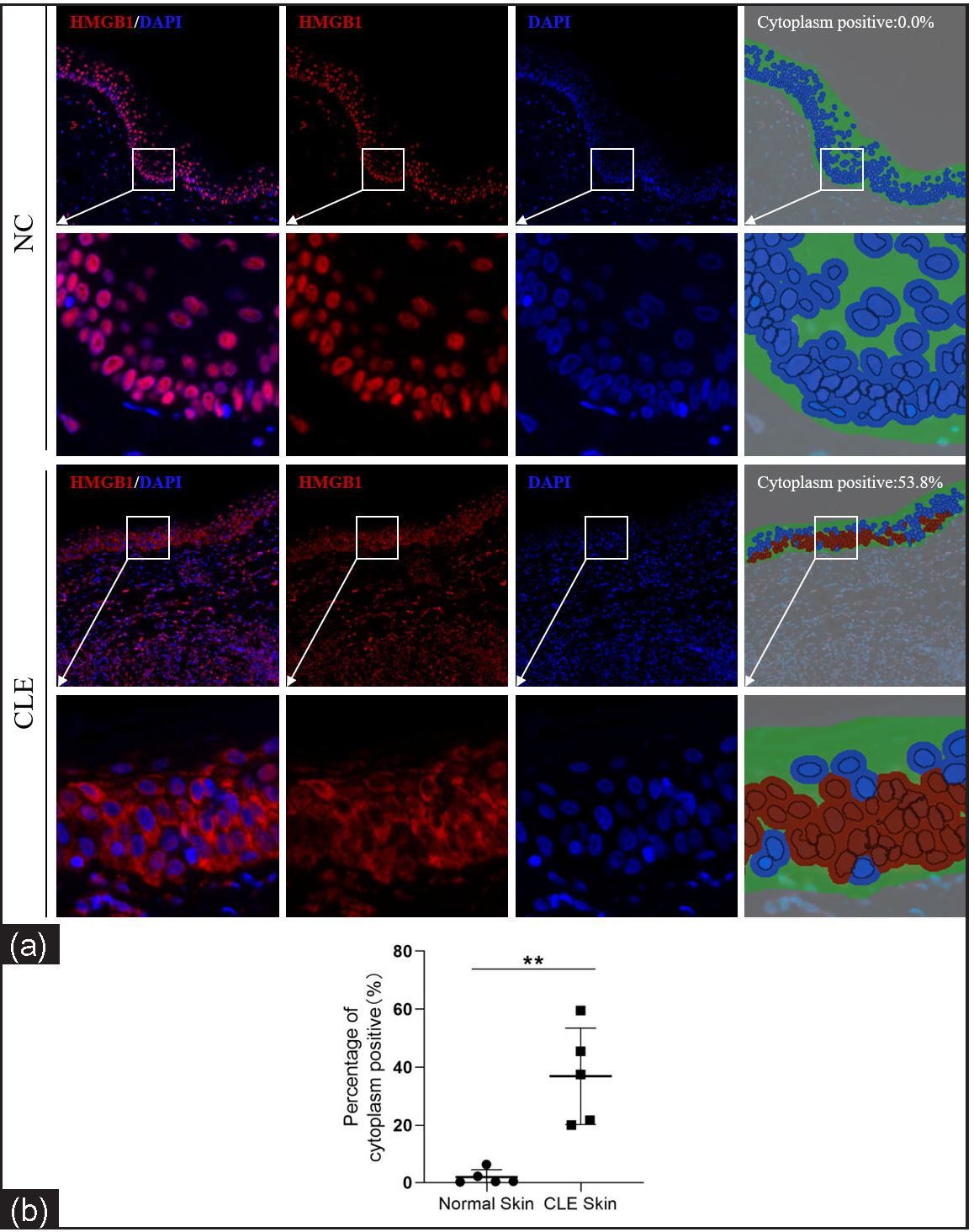
- Nuclear-cytoplasmic localization expression of HMGB1 in the epidermis of CLE patients (n = 5) and healthy controls (n = 5; IHF, 200×). HMGB1 staining is shown in red and nuclear DAPI staining is shown in blue. The irregular green part of cytoplasm positive indicates the epidermis and the red circle indicates the HMGB1 expression localised in the cytoplasm after analysis using Inform software (**P < 0.01).
HMGB1 levels in the keratinocytes treated with the SIRT1 activator Res and UVB radiation
We analysed the HMGB1 levels in HaCaT cells treated with Res (0 and 10 µM) and then irradiated by UVB (0 and 30 mJ/cm2) using RT-qPCR and western blotting. The nuclear-cytoplasmic localization expression of HMGB1 was detected using immunocytofluorescence. In contrast to the control group (without Res treatment), the nuclear protein expression of HMGB1 was significantly increased in the experimental group (with 10 µM Res treatment; 0 mJ/cm2, 0 µM Res vs. 0 mJ/cm2, 10 µM Res: 0.75 ± 0.06 vs. 1.03 ± 0.12, P = 0.020, n = 3; 30 mJ/cm2, 0 µM Res vs. 30 mJ/cm2, 10 µM Res: 0.83 ± 0.07 vs. 1.02 ± 0.02, P = 0.011, n = 3; Figure 3). The cytoplasmic protein expression of HMGB1 was significantly decreased in the experimental group (with 10 µM Res treatment) compared to the control group (without Res treatment; 0 mJ/cm2, 0 µM Res vs. 0 mJ/cm2, 10 µM Res: 0.98 ± 0.14 vs. 0.76 ± 0.02, P = 0.048, n = 3; 30 mJ/cm2, 0 µM Res vs. 30 mJ/cm2, 10 µM Res: 1.07 ± 0.06 vs. 0.62 ± 0.17, P = 0.012, n = 3; Figure 3). No significant differences were found in the mRNA and total protein expression levels of HMGB1 between the groups (P > 0.05; Figure 3).
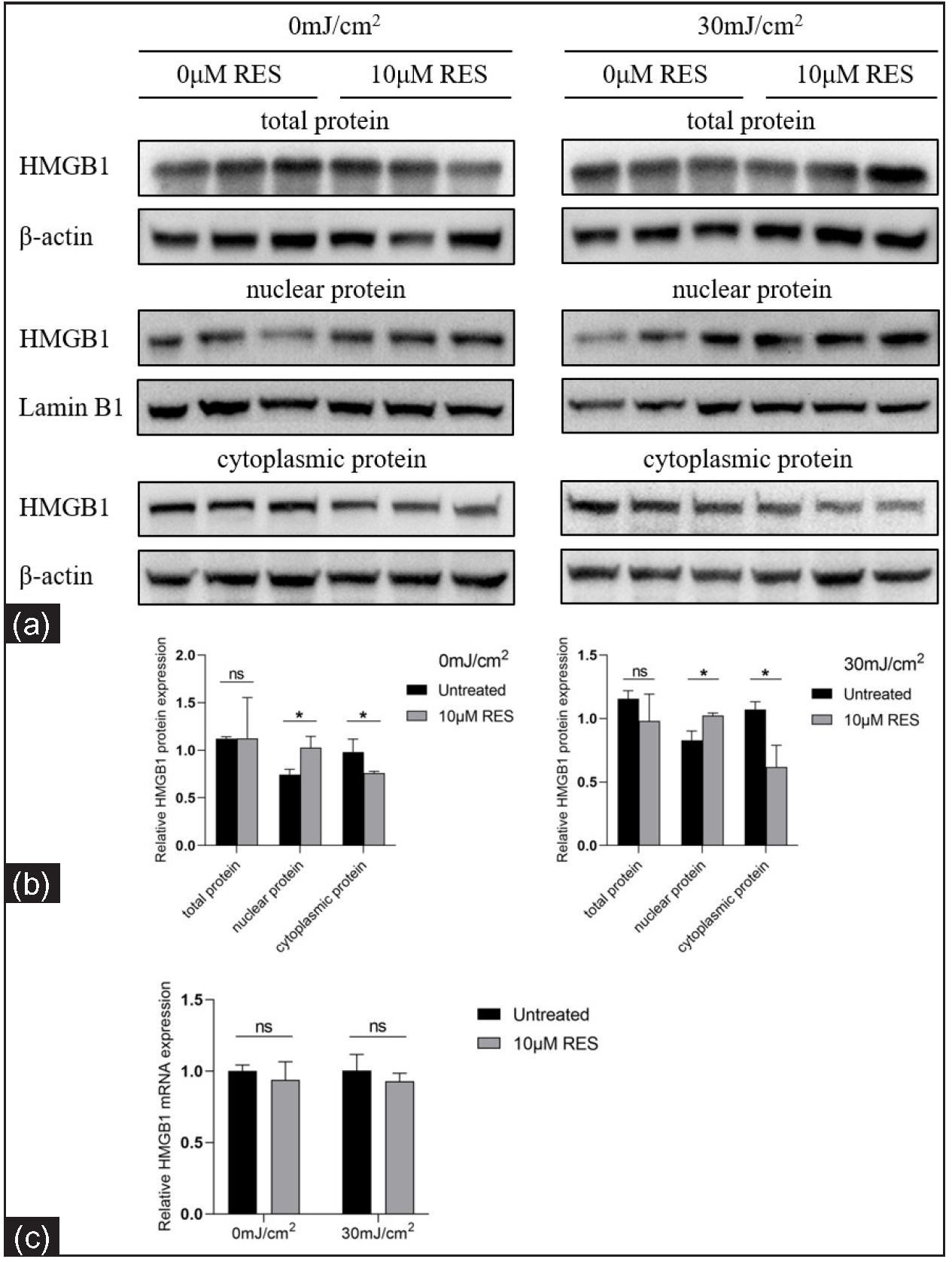
- Relative mRNA and protein levels of HMGB1 in keratinocytes treated with the SIRT1 activator Res and UVB radiation (n = 3). (a, b) Comparison of HMGB1 protein levels in HaCaT cells treated with the SIRT1 activator Res (0 and 10 µM) and UVB radiation (0 and 30 mJ/cm2). (c) Comparison of HMGB1 mRNA levels in HaCaT cells treated with the SIRT1 activator Res (0 and 10 µM) and UVB irradiation (0 and 30 mJ/cm2) (n = 4) (*P < 0.05, ns P > 0.05).
Compared to the control group (0 mJ/cm2, 0 µM Res), the cytoplasmic positivity rate (%) of HMGB1 was significantly higher in the experimental group (30 mJ/cm2, 0 µM Res; 6.94 ± 2.57 vs. 13.40 ± 4.54, P = 0.002, n = 3; Figure 4). Compared to the control group (without Res treatment), the cytoplasmic positivity rate (%) of HMGB1 was significantly lower in the experimental group (with 10 µM Res treatment; 0 mJ/cm2, 0 µM Res vs. 0 mJ/cm2, 10 µM Res: 6.94 ± 2.57 vs. 3.51 ± 2.31, P = 0.009, n = 3; 30 mJ/cm2, 0 µM Res vs. 30 mJ/cm2, 10 µM Res: 13.40 ± 4.54 vs. 4.75 ± 2.34, P < 0.001, n = 3; Figure 4). The results of nuclear DAPI immunofluorescence showed that the nuclei of early apoptotic cells were condensed and stained darker, whereas the nuclei of late apoptotic cells were cleaved. HMGB1 translocation was associated with the apoptosis of cells.
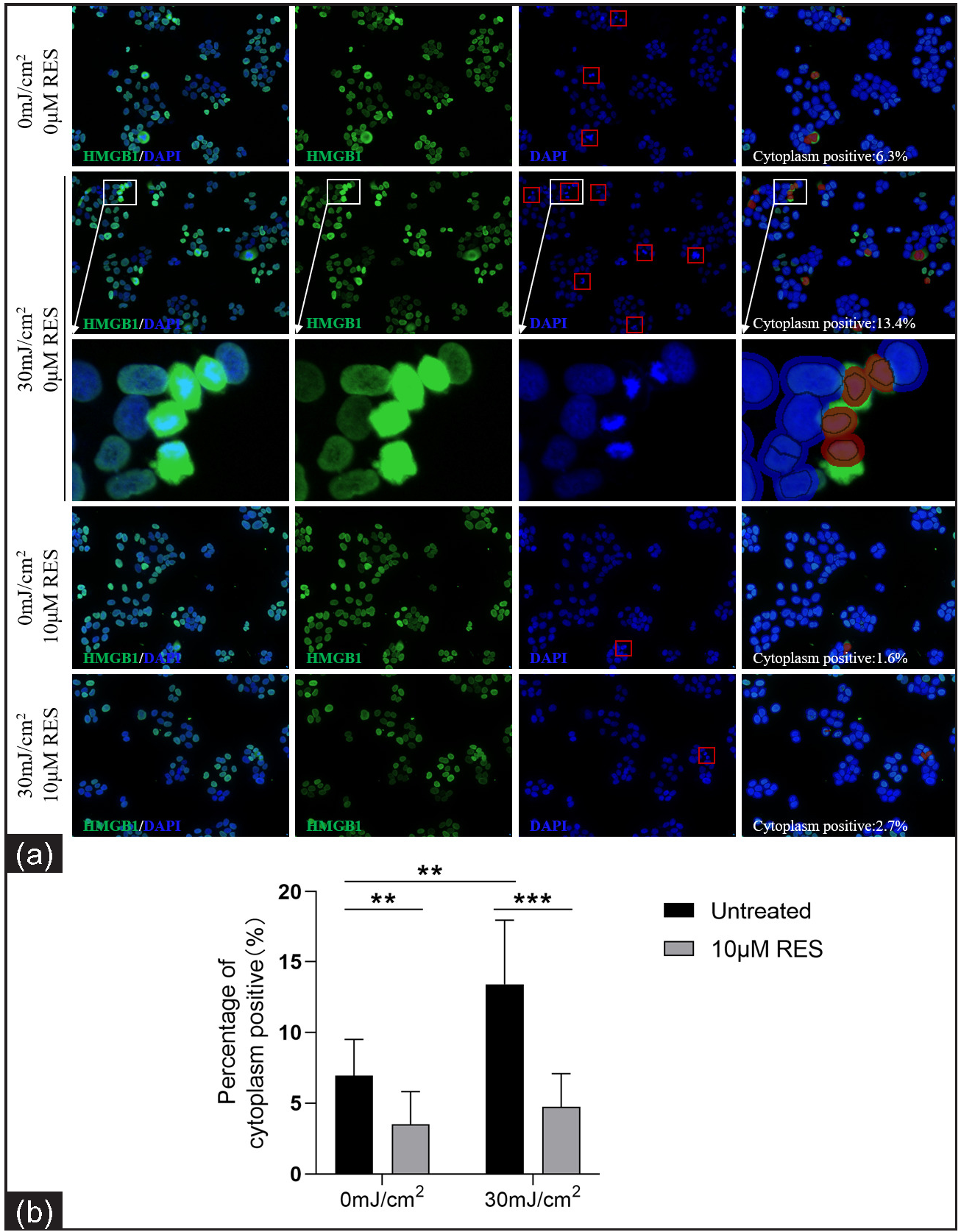
- Nuclear-cytoplasmic localization expression of HMGB1 in HaCaT cells treated with the SIRT1 activator Res (0 and 10 µM) and UVB radiation (0 and 30 mJ/cm2) (n = 3; ICF, 200×). HMGB1 staining is shown in green and nuclear DAPI staining is shown in blue. The red box in the DAPI stained image indicates apoptotic cells. The red circle in cytoplasm positive indicates HMGB1 expression localised in the cytoplasm after analysis using Inform software (**P < 0.01, ***P < 0.001).
Acetyl-HMGB1 level in keratinocytes treated with the SIRT1 activator Res and UVB radiation
We detected the acetyl-HMGB1 level in HaCaT cells treated with Res (0 and 10 µM) and then irradiated by UVB (0 and 30 mJ/cm2) using IP. Compared to the control group (without Res treatment), the acetyl-HMGB1 level was significantly lower in the experimental group (with 10 µM Res treatment; 0 mJ/cm2, 0 µM Res vs. 0 mJ/cm2, 10 µM Res: 0.93 ± 0.15 vs. 0.64 ± 0.04, P =0.032, n = 3; 30 mJ/cm2, 0 µM Res vs. 30 mJ/cm2, 10 µM Res: 1.05 ± 0.14 vs. 0.75 ± 0.06, P = 0.027, n = 3; Figure 5).
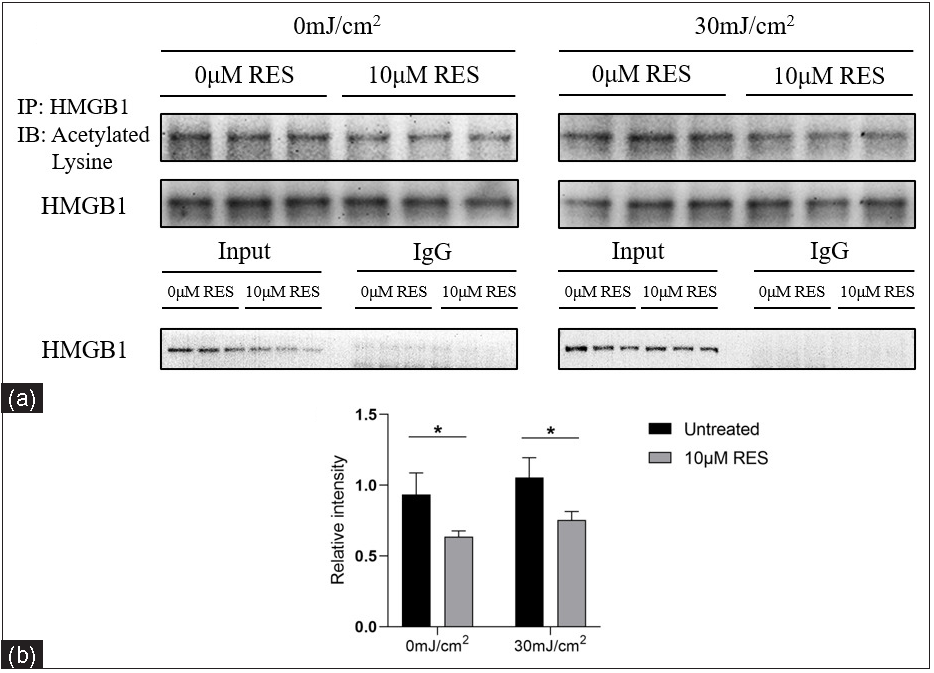
- Acetyl-HMGB1 level in HaCaT cells treated with the SIRT1 activator Res (0 and 10 µM) and UVB radiation (0 and 30 mJ/cm2) (n = 3). IP analysis showed that compared to the control group (without Res treatment), the acetyl-HMGB1 level was significantly decreased in the experimental group (with 10 µM Res treatment) (*P < 0.05).
Apoptosis level and cell cycle proportion of keratinocytes
The apoptosis level and cell cycle proportion of NHEK cells treated with Res (0 and 10 µM) and then irradiated by UVB (0 and 30 mJ/cm2) were detected using flow cytometry. Compared to the control group (without Res treatment), the apoptosis rate (%) in the experimental group (with 10 µM Res treatment) was significantly lower (0 mJ/cm2, 0 µM Res vs. 0 mJ/cm2, 10 µM Res: 49.72 ± 5.49 vs. 21.49 ± 2.20, P = 0.003, n = 3; 30 mJ/cm2, 0 µM Res vs. 30 mJ/cm2, 10 µM Res: 41.85 ± 2.26 vs. 24.07 ± 2.97, P = 0.001, n = 3; Figures 6a–6c). Compared to the control group (without Res treatment), the proportion of cells in the S phase (%) was significantly higher in the experimental group (with 10 µM Res treatment; 0 mJ/cm2, 0 µM Res vs. 0 mJ/cm2, 10 µM Res: 44.00 ± 0.66 vs. 47.81 ± 1.41, P = 0.013, n = 3; 30 mJ/cm2, 0 µM Res vs. 30 mJ/cm2, 10 µM Res: 31.06 ± 1.55 vs. 35.88 ± 1.21, P = 0.013, n = 3). No significant differences were found in the proportion of cells in the G0/G1 and G2/M phases (P > 0.05, n = 3; Figures 6d–6f).
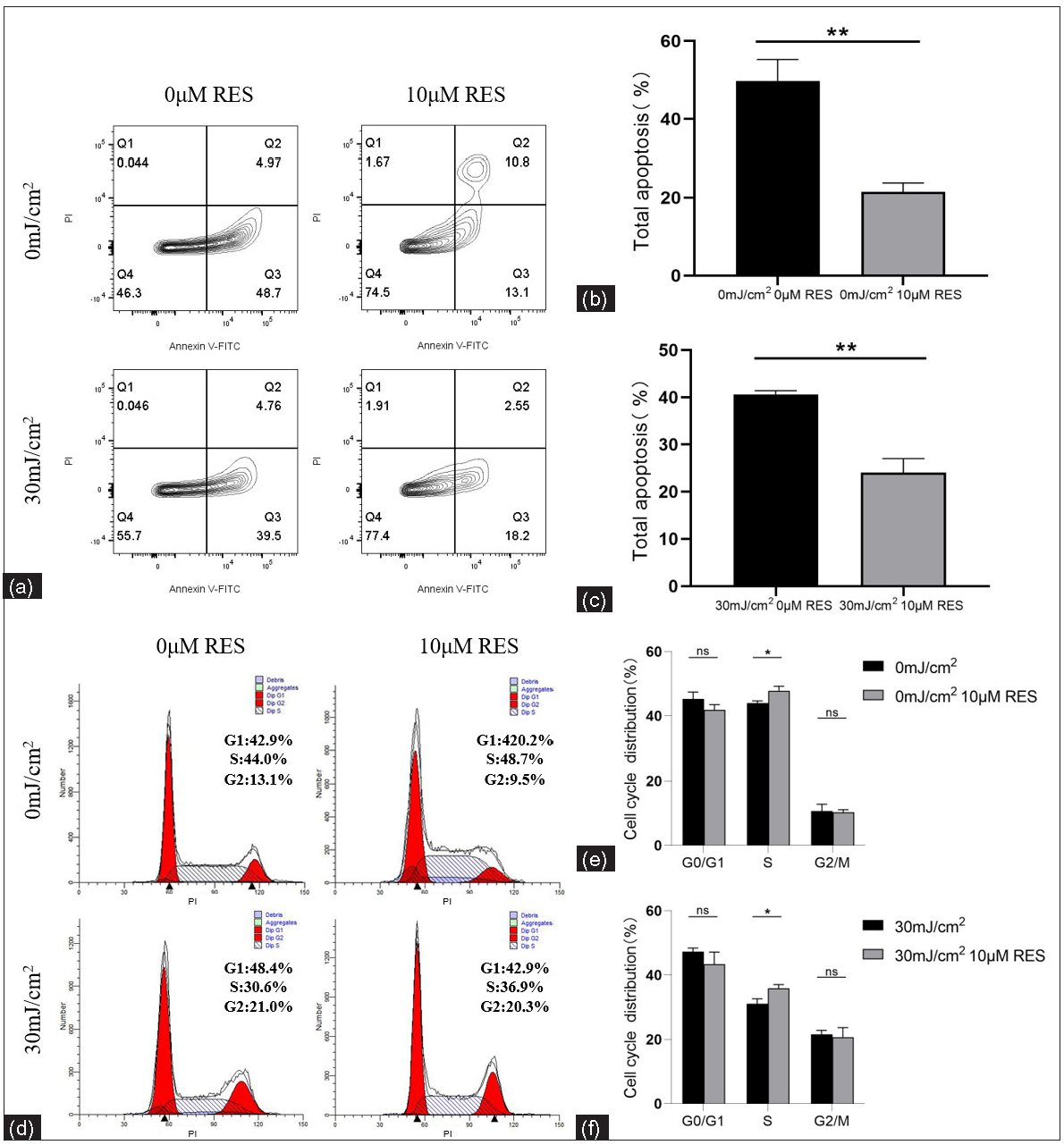
- Apoptosis levels and cell cycle proportion of keratinocytes. (a, b) Comparison of apoptosis levels of NHEK cells treated with Res (0 and 10 µM) and then irradiated by UVB (0 mJ/cm2) (n = 3). (a, c) Comparison of apoptosis levels of NHEK cells treated with Res (0 and 10 µM) and then irradiated by UVB (30 mJ/cm2) (n = 3). (d, e) Comparison of cell cycle proportion in NHEK cells treated with Res (0 and 10 µM) and then irradiated by UVB (0 mJ/cm2) (n = 3). (d, f) Comparison of cell cycle proportion in NHEK cells treated with Res (0 and 10 µM) and then irradiated by UVB (30 mJ/cm2) (n = 3) (*P < 0.05, **P < 0.01, ns P > 0.05).
Discussion
Skin damage is the main clinical manifestation of CLE13 and UV radiation can lead to CLE progression.14 UV irradiation can trigger inflammatory responses in the skin through multiple mechanisms, including keratinocyte apoptosis and release of autoantigens, pro-inflammatory factors and HMGB1.4 Nuclear HMGB1 binds to damaged DNA and recruits various DNA repair proteins and chromatin remodelling factors to promote DNA damage repair.15 Cells actively release HMGB1 in response to pro-inflammatory stimuli.16 In addition, cells experiencing non-programmed death also passively secrete HMGB1, thereby promoting an inflammatory response.16
Our previous research reported that the HMGB1 mRNA level in CD4+ T cells of SLE patients was positively correlated with systemic lupus erythematosus disease activity index (SLEDAI).17 Other studies have reported that the serum level of HMGB1 was elevated in SLE patients and related to SLEDAI.18 The increase in the level of circulating HMGB1 in SLE patients may lead to immune complex formation.19
HMGB1 acetylation is closely related to its nuclear and cytoplasmic translocation, secretion and release. As an HDAC, SIRT1 regulates the deacetylation of histone and non-histone proteins, such as HMGB1, and plays a crucial role in the regulation of various physiological processes.11,20 The SIRT1 mRNA and protein levels in CD4+ T cells of SLE patients were remarkably reduced after UVB irradiation.2 Activation and proliferation of CD4+ T cells and peripheral blood mononuclear cells are suppressed in SLE patients after treatment with the SIRT1 activator Res.2 The reduced SIRT1 expression level correlates with the SLE disease activity.2 Our results demonstrated that SIRT1 level was significantly decreased in the epidermis of CLE patients. Furthermore, a similar phenomenon was observed in keratinocytes after UVB irradiation, suggesting that the decreased SIRT1 expression may be associated with CLE and UV-induced skin lesions in LE.
We found that the acetyl-HMGB1 level was increased in keratinocytes after UVB irradiation, leading to HMGB1 translocation. Nuclear HMBG1 can bind to DNA and repair the UV-induced DNA damage through the NER pathway.7,21 Therefore, we speculate that HMGB1 translocation from the nucleus to the cytoplasm leads to a relative deficiency of nuclear HMGB1 which promotes DNA repair defects and leads to the development of LE lesions.
Treatment with the SIRTl activator Res was associated with reduced acetyl-HMGB1 level and HMGB1 translocation in keratinocytes. Other studies reported that Res treatment was associated with a significantly reduced acetyl-HMGB1 level in the damaged area of the cerebral cortex after traumatic brain injury. In addition, HMGB1 translocation was inhibited.22 The Lys site at which SIRT1 binds to HMGB1 and induces its deacetylation differs between different cell lines. In mouse mononuclear macrophages RAW264.7, the action sites of SIRT1 on HMGB1 deacetylation are Lys28–30,12 while in human umbilical vein cells EA.hy926, the action sites are Lys55, 88, 90 and 177.23 Further studies are needed to identify the Lys site that is affected by the SIRT1 deacetylation of HMGB1 in keratinocytes.
Apoptosis induces autoantigen production in SLE, promotes the binding of autoantibodies to antigens and activates the abnormal immune responses.24 Studies have shown that high concentrations of HDAC inhibitors may induce apoptosis.24 Our study found that the SIRT1 activator Res reduces the apoptosis level of keratinocytes and protects against UV-induced apoptosis. It was reported that HMGB1 was released from necrotic cells into the extracellular space in cholesteatoma.25 In infectious inflammatory diseases, HMGB1 can promote proliferation and reduce apoptosis in keartinocytes by inducing the activation of RAGE and Akt signalling pathways and causing the translocation of NF-κB p65.25 In our study, HMGB1 translocation occurred in apoptotic keratinocytes and activated HMGB1 promoted apoptosis in autoimmune inflammatory diseases. The different types of inflammation may have different mechanisms. Whether HMGB1 promotes or inhibits apoptosis, it ultimately promotes the development of inflammation and the molecular mechanisms need to be further investigated.
UV irradiation can also initiate cell senescence. Senescent cells undergo changes in signalling transduction pathways. Bric-a-Bric (BTB) and Cap’n’ collar (CNC) homology 2 (Bach2), a highly sensitive DNA damage response factor, is downregulated during DNA damage and cell senescence.26 UV irradiation downregulates Bach2 in skin fibroblasts, activating the p53 and p16INK4a pathways to prevent cell growth.27 At present, there are no relevant studies on the role of Bach2 in irradiated and lupus keratinocytes which provides a new direction for further research.
Limitations
In our study, we only treated keratinocytes with the SIRT1 activator but did not perform the relevant experiments in keratinocytes with SIRT1 knockdown or overexpression. In addition to activating SIRT1, Res has a wide range of biological effects, such as antioxidant and immunomodulatory effects, while gene overexpression is achieved via plasmid transfection for massive transcription and translation, resulting in overexpression of the gene product. Moreover, the Lys site of action of SIRT1 deacetylation of HMGB1 is unclear. The specific mechanism of action of SIRT1 deacetylation of HMGB1 needs to be further investigated.
Conclusion
We found that SIRT1 expression was decreased and HMGB1 was translocated from the nucleus to the cytoplasm in the epidermis of CLE patients and UVB-irradiated keratinocytes. In keratinocytes, SIRT1 inhibits nuclear HMGB1 translocation to the cytoplasm induced by UVB irradiation through HMGB1 deacetylation, thereby suppressing apoptosis. This study elucidated the crucial role and mechanisms of HMGB1 translocation in keratinocytes in the development of lupus erythematosus lesions, providing a new direction for the study of pathogenesis and a new potential target for treatment. Future research should focus on the detailed molecular mechanisms underlying SIRT1-induced HMGB1 deacetylation in CLE.
Declaration of patient consent
The authors certify that they have obtained all appropriate patient consent.
Financial support and sponsorship
The National Natural Science Foundation of China (NO: 81472881). The Natural Science Foundation of Changsha, Hunan, China (NO: kq2208323)
Conflicts of interest
There are no conflicts of interest.
References
- DNA double-strand breaks: Their production, recognition, and repair in eukaryotes. Mutat Res. 2009;669:8-12.
- [CrossRef] [PubMed] [Google Scholar]
- Ultraviolet B inhibition of DNMT1 activity via AhR activation dependent SIRT1 suppression in CD4+ T cells from systemic lupus erythematosus patients. J Dermatol Sci. 2017;86:230-7.
- [CrossRef] [PubMed] [Google Scholar]
- Apoptosis of keratinocytes and serum DNase I activity in patients with cutaneous lupus erythematosus: relationship with clinical and immunoserological parameters. J Eur Acad Dermatol Venereol. 2017;31:523-9.
- [CrossRef] [PubMed] [Google Scholar]
- The extracellular release of HMGB1 during apoptotic cell death. Am J Physiol Cell Physiol. 2006;291:C1318-1325.
- [CrossRef] [PubMed] [Google Scholar]
- Clearance of apoptotic cells in human SLE. Curr Dir Autoimmun. 2006;9:173-87.
- [CrossRef] [PubMed] [Google Scholar]
- HMGB1 in systemic lupus erythematosus. Front Immunol. 2020;11:1057.
- [CrossRef] [PubMed] [PubMed Central] [Google Scholar]
- High-mobility group box 1 protein (HMGB1): nuclear weapon in the immune arsenal. Nat Rev Immunol. 2005;5:331-42.
- [CrossRef] [PubMed] [Google Scholar]
- Extracellular HMGB1 as a therapeutic target in inflammatory diseases. Expert Opin Ther Targets. 2018;22:263-77.
- [CrossRef] [PubMed] [Google Scholar]
- Emerging roles for HMGB1 protein in immunity, inflammation, and cancer. Immunotargets Ther. 2015;4:101-109.
- [CrossRef] [PubMed] [PubMed Central] [Google Scholar]
- Redox regulation of SIRT1 in inflammation and cellular senescence. Free Radic Biol Med. 2013;61:95-110.
- [CrossRef] [PubMed] [PubMed Central] [Google Scholar]
- Characterization of FOXO acetylation. Methods Mol Biol. 2019;1890:77-90.
- [CrossRef] [PubMed] [Google Scholar]
- Ethyl pyruvate inhibits the acetylation and release of HMGB1 via effects on SIRT1/STAT signaling in LPS-activated RAW264.7 cells and peritoneal macrophages. Int Immunopharmacol. 2016;41:98-105.
- [CrossRef] [PubMed] [Google Scholar]
- Immunogenetics of cutaneous lupus erythematosus. Curr Opin Pediatr. 2016;28:470-75.
- [CrossRef] [PubMed] [PubMed Central] [Google Scholar]
- Cutaneous lupus erythematosus: An update on pathogenesis, diagnosis and treatment. Am J Clin Dermatol. 2016;17:135-46.
- [CrossRef] [PubMed] [Google Scholar]
- Interactions of high mobility group box protein 1 (HMGB1) with nucleic acids: Implications in DNA repair and immune responses. DNA Repair (Amst). 2019;83:102701.
- [CrossRef] [PubMed] [PubMed Central] [Google Scholar]
- Release of chromatin protein HMGB1 by necrotic cells triggers inflammation. Nature. 2002;418:191-5.
- [CrossRef] [PubMed] [Google Scholar]
- A possible role of HMGB1 in DNA demethylation in CD4+ T cells from patients with systemic lupus erythematosus. Clin Dev Immunol. 2013;2013:206298.
- [CrossRef] [PubMed] [PubMed Central] [Google Scholar]
- Serum high-mobility group box 1 is correlated with interferon-α and may predict disease activity in patients with systemic lupus erythematosus. Lupus. 2019;28:1120-7.
- [CrossRef] [PubMed] [Google Scholar]
- Translocation of the novel cytokine HMGB1 to the cytoplasm and extracellular space coincides with the peak of clinical activity in experimentally UV-induced lesions of cutaneous lupus erythematosus. Lupus. 2007;16:794-802.
- [CrossRef] [PubMed] [Google Scholar]
- SIRT1-dependent regulation of chromatin and transcription: linking NAD(+) metabolism and signaling to the control of cellular functions. Biochim Biophys Acta. 2010;1804:1666-75.
- [CrossRef] [PubMed] [PubMed Central] [Google Scholar]
- HMGB1 interacts with XPA to facilitate the processing of DNA interstrand crosslinks in human cells. Nucleic Acids Res. 2016;44:1151-60.
- [CrossRef] [PubMed] [PubMed Central] [Google Scholar]
- Omega-3 polyunsaturated fatty acid attenuates the inflammatory response by modulating microglia polarization through SIRT1-mediated deacetylation of the HMGB1/NF-κB pathway following experimental traumatic brain injury. J Neuroinflammation. 2018;15:116.
- [CrossRef] [PubMed] [PubMed Central] [Google Scholar]
- High-mobility group box 1 is a novel deacetylation target of Sirtuin1. Kidney Int. 2015;87:95-108.
- [CrossRef] [PubMed] [PubMed Central] [Google Scholar]
- Cell death in the pathogenesis of systemic lupus erythematosus and lupus nephritis. Clin Immunol. 2017;185:59-73.
- [CrossRef] [PubMed] [PubMed Central] [Google Scholar]
- Molecular signaling of the HMGB1/RAGE axis contributes to cholesteatoma pathogenesis. J Mol Med (Berl). 2015;93:305-314.
- [CrossRef] [PubMed] [PubMed Central] [Google Scholar]
- BACH2: A marker of DNA damage and ageing. DNA Repair (Amst). 2013;12:982-992.
- [CrossRef] [PubMed] [PubMed Central] [Google Scholar]
- Bach2 regulates autophagy to modulate UVA-induced photoaging in skin fibroblasts. Free Radic Biol Med. 2021;169:304-16.
- [CrossRef] [PubMed] [Google Scholar]