Translate this page into:
Intracutaneous pharmacokinetics of oral antifungals and their relevance in recalcitrant cutaneous dermatophytosis: Time to revisit basics
2 Department of Dermatology, Hindu Rao Hospital, New Delhi, India
Correspondence Address:
Pooja Arora
Dr. Ram Manohar Lohia Hospital and PGIMER, New Delhi
India
How to cite this article: Sardana K, Arora P, Mahajan K. Intracutaneous pharmacokinetics of oral antifungals and their relevance in recalcitrant cutaneous dermatophytosis: Time to revisit basics. Indian J Dermatol Venereol Leprol 2017;83:730-732 |
Introduction
The recent upsurge in the incidence of chronic and relapsing dermatophyte infections have led to increased efforts to enable effective interventions, which mostly focus on pharmacological up-dosing of antifungal agents and seeking various combinations, based on little in-vitro data or understanding of the complex pathogenesis of the infection. It must be noted that the information provided by standard antifungal susceptibility test (AFST) methods; the MIC (minimum inhibitory concentration) or the disk zone diameter, may not always have clinical relevance in the care of patients with fungal infection.[1] It is this issue (the “clinical utility” or “clinical relevance” of AFST) that is rarely discussed. An important paper had articulated several key principles to consider when discussing the clinical utility of susceptibility test methods.[1] These principles include an understanding that the MIC is a construct that is largely defined by testing conditions, rather than a physical or chemical measurement. This measure might correlate with the clinical outcome, but a multitude of factors related to the host (immune response, underlying illness, site of infection), the infecting organism (virulence) and the antifungal agent [dose, pharmacokinetics (PK), pharmacodynamics (PD), drug interactions] may be more important than susceptibility test results in determining clinical outcomes for infected patients.[2] In particular, in-vitro susceptibility of an organism to an antifungal agent does not consistently predict a successful therapeutic outcome.[2]
Another concept in relation to antifungal agents is the epidemiological cut off value (ECV) which represents the MIC value that separates microbial populations into those with and without acquired or mutational resistance, based on their phenotypes. The ECV defines the upper limit of susceptibility for the wild-type population of the microbe and is solely based on in-vitro laboratory data collected from multiple laboratories and cannot be used by itself to predict clinical outcome of therapy.
Pharmacokinetics/Pharmacodynamics of Systemic Antifungals
Of all, systemic antifungals still form the bulwark of interventions. Two major features of an antifungal agent determine its therapeutic efficacy in dermatomycosis: the direct antifungal effect, usually expressed in minimal inhibitory concentration (MIC) values and its availability at the site of infection.[3] The latter is crucial as the focus is the skin or more correctly, the stratum corneum.[4] An additional dimension of antimicrobial pharmacodynamics (PD) revolves around the drug exposure as relative to a measure of MIC. Three traditional PD parameters have been used to describe the relationship of drug and dosage. These include the peak concentration in relation to the MIC (Cmax/MIC), the area under the concentration curve in relation to the MIC [24 h area under the concentration curve area under the curve (AUC)/MIC], and the time that drug concentrations exceed the MIC expressed as a percentage of the dosing interval (%T > MIC) [Figure - 1]. To further choose the drug, two outcomes are commonly noted. The first is the impact of increasing drug concentrations on the rate and extent of organism killing, called the concentration dependent effect. The second study endpoint includes examination of antifungal activity after drug concentrations decrease below the organism MIC. For some drugs, there is a period of prolonged growth suppression following an initial supra-MIC exposure. This period of growth suppression is termed as post-antifungal effect (PAFE).[4],[5] Three combinations of these time kill endpoint characteristics have been observed and each combination is predictive of one of the PD parameters. The Cmax/MIC is associated with concentration-dependent killing and prolonged PAFEs. The %T >MIC is associated with concentration-independent killing and short PAFEs. The AUC/MIC is associated with prolonged PAFEs and either concentration-dependent or -independent killing. The time kill combination of concentration-independent killing and prolonged PAFEs suggest that the 24th AUC/MIC parameter is most closely tied to treatment effect for triazoles. Furthermore, this measure depends on AUC of serum levels which do not reflect the levels in the skin.[6] Importantly, most in-vitro data tend to correlate the serum AUC levels with skin MIC data, which are not comparable.
![]() |
Figure 1: Diagrammatic representation of pharmacokinetics/pharmacodynamics of oral antifungal drugs |
The levels of various oral antifungals in the skin depend on the route of delivery of antifungals and can be via sebum, sweat, keratin, or via diffusion through the dermis.[7] This has relevance as these may have site and age dependent variations. Because the infection is largely localized to the human stratum corneum alone, it would be relevant to focus on studies that have studied the retention time of the active drug in the horny layer.[7],[8],[9] This also highlights the major drawback of susceptibility tests which do not take into account various other factors including cutaneous levels of the drug and host immune response, and hence do not correlate consistently with in-vivo efficacy.[6],[7] Infact, data regarding MIC vary substantially depending on experimental conditions and do not reflect the activity of the drug in target human tissues.[6],[7],[8]
The serum levels of oral antifungals do not parallel the cutaneous levels, with levels of drugs varying from 10 to 73 times the values in the serum. These levels depend on the class of drugs used which in turn predicts the route of delivery.[8],[9],[10],[11],[12]
Clinical Relevance of Skin Pharmacology of Systemic Antifungals
As seen in [Table - 1], itraconazole (ITR) and terbinafine (TER) are lipophilic and their route of distribution is dependent on sebum. Thus, in sites where the sebum excretion is minimal like the palms and soles or in patients with marked xerosis these two drugs may not be effective. Thus, for example the levels of ITR in the palms are relatively low (incorporation in basal layer) but they persist at least 3 weeks after the end of therapy because of the thickness of the stratum corneum.[6],[10] The back has a rather thin stratum corneum with an even distribution of sebum and sweat glands. This results in levels higher than the corresponding plasma levels, but in the disappearance of the drug within 2 weeks after the end of therapy (parallel with disappearance of detectable sebum and sweat levels).[6],[10] The beard region has a much higher level of sebum excretion. As a consequence, ITR levels are extremely high and therefore remain measurable up to 4 weeks after discontinuation of therapy. It binds tightly in the stratum corneum and does not readily redistribute to the plasma compartment, accounting for its persistence.[6],[10] The same principles hold true for TER. These studies have also shed light on the dosimetry and the closest to a complete fungicidal effect was observed with 200 mg ITR twice daily against Trichophyton mentagrophytes.[10] Though TER was comparable to 200 mg/day ITR as an antidermatophyte drug, a dose of ITR of 200 mg twice a day had a faster onset of action than TER.[10] Here it must be emphasized that with ITR, giving a higher dose does not translate consistently into a higher antifungal effect as the drug has nonlinear PK and increasing the dose does not translate to higher serum levels.
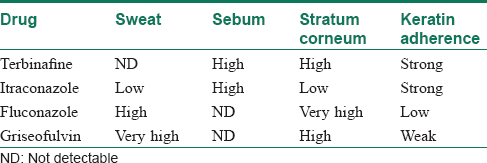
Thus, in situ ations where either the site of infection has less sebum or the patient has an intrinsically dry skin or in case of clinical failures without resistance, fluconazole (FLU) may be the ideal drug, as it has the highest levels in the stratum corneum [Table - 1]. It is suggested that a high concentration of an antifungal agent is required in the stratum corneum and epidermis–dermis junction for efficacy against dermatophytosis.[5] FLU is preferentially localized to both the stratum corneum and epidermis-cutis at high concentrations.[10],[11],[12] It directly diffuses into the epidermis-cutis from capillary blood vessels, and a major portion of it exists as a nonbinding form (because of its small extent of binding to corneous keratin).[10],[11],[12] Thus, it may be useful in situ ations where other anti fungals fail, but it must be emphasized that this is with a daily dose and not the weekly dose (50mg/d for 12 days). This is because the levels achieved in the stratum corneum was the highest for daily FLU (66.4μ(6gm) as compared to weekly FLU (23.4 ag/gm).[11]
Thus, the clinical results obtained with ITR and TER indicate that in-vitro and animal model data cannot predict clinical outcome, which depends on the interplay between infecting strain, host defense, epidermal turnover, pharmacokinetic properties and intrinsic antimycotic activity of the drug. Individual differences among patients, and the local immunity is of paramount importance, a topic that is beyond the scope of this article.[13]
In a study done by Miskeen AK et al., griseofulvin was found to be an effective drug against dermatophytes as cultures tested were susceptible to the drug which had MICs less than 2μg/ml.[14] However, other studies have found a high MIC value for griseofulvin. Also, this drug may not be so effective for treatment of dermatophytosis due to its PK and excretion in skin.
Importantly, the other drugs like voriconazole, amphotericin B, anidulafungin and caspofungin do not achieve the same concentration in the skin as compared to that in the plasma and would hence unlikely to be of use for dermatophytosis.[3],[4],[5]
Summary and Conclusions
The misinterpretation of the finer nuances of PK and an eagerness to treat the dermatophytic infections at the earliest, have forced the clinicians to use alternative therapies like retinoids in combination with antifungals.[15] However, retinoids markedly reduce the sebum production which affects the levels of ITR and TER drugs that are largely lipophilic.[16] Further in-vivo studies need to be carried out in this direction.
Thus, on the basis of cutaneous PK/PD studies, some useful clinical interpretations can be arrived at:
- The lipophilicity of ITR and TER makes them useful in infections on the face and trunk, but may not be useful on the palms and soles and in extremes of ages or patients with xerosis.[5],[6],[7]
- The pharmacological skin PK secretion precludes a combination of isotretinoin with ITR and TER as these antifungal drugs are lipophilic [3],[4],[5],[6],[7],[10],[16] and will achieve little cutaneous levels with isotretinoin.
- PK studies show that ITR 200 has a faster onset of action than 100 mg and in a dose of 200 mg twice a day may be better than TER 250 mg once a day for dermatophytoses.[6],[7],[10]
- In cases of clinical failure on ITR and TER, it would be logical to administer FLU based on the very high levels attained by FLU in the stratum corneum, but in a daily dose of FLU 50 mg for12 days.[11],[12]
- The keratin adherence of TER and ITR indicates that their effects last for 3 weeks in the skin, thus a relapse can be defined as a recurrence of infection after 3 weeks, but again it would depend on the body site affected.
Most importantly, to make any clinical utility of in-vitro MIC data, these should be correlated with the levels of the antifungal drugs in the skin and not in the serum. As these drugs can achieve very high levels in the skin as compared to the plasma, we believe that persistent and recalcitrant dermatophytoses, may possibly be explained by other factors, linked to host immunity, than the often cited failure of antifugal drugs due to loss of intrinsic efficacy or in-vitro resistance to systemic anti fungal (AF) drugs.[13],[16]
Financial support and sponsorship
Nil.
Conflicts of interest
There are no conflicts of interest.
1. |
Rex JH, Pfaller MA. Has antifungal susceptibility testing come of age? Clin Infect Dis 2002;35:982-9.
[Google Scholar]
|
2. |
Diekema DJ, Pfaller MA. Utility of antifungal suceptibility testing and clinical correlations. In: Hall GS, editor. Interactions of Yeasts, Moulds, and Antifungal Agents: How to Detect Resistance. 1st ed. New York: Springer; 2012. p. 131-58.
[Google Scholar]
|
3. |
Andes D. Clinical utility of antifungal pharmacokinetics and pharmacodynamics. Curr Opin Infect Dis 2004;17:533-40.
[Google Scholar]
|
4. |
Andes D. Pharmacokinetics and pharmacodynamics of antifungals. Infect Dis Clin North Am 2006;20:679-97.
[Google Scholar]
|
5. |
De Doncker P. Pharmacokinetics of oral antifungal agents. Dermatol Ther 1997;3:46-57.
[Google Scholar]
|
6. |
Cauwenbergh G, Degreef H, Heykants J, Woestenborghs R, Van Rooy P, Haeverans K. Pharmacokinetic profile of orally administered itraconazole in human skin. J Am Acad Dermatol 1988;18 (2 Pt 1):263-8.
[Google Scholar]
|
7. |
Piérard GE, Piérard-Franchimont C, Estrada JA. Comparative study of the activity and lingering effect of topical antifungals. Skin Pharmacol 1993;6:208-14.
[Google Scholar]
|
8. |
Faergemann J, Zehender H, Denouël J, Millerioux L. Levels of terbinafine in plasma, stratum corneum, dermis-epidermis (without stratum corneum), sebum, hair and nails during and after 250 mg terbinafine orally once per day for four weeks. Acta Derm Venereol 1993;73:305-9.
[Google Scholar]
|
9. |
Faergemann J, Zehender H, Jones T, Maibach I. Terbinafine levels in serum, stratum corneum, dermis-epidermis (without stratum corneum), hair, sebum and eccrine sweat. Acta Derm Venereol 1991;71:322-6.
[Google Scholar]
|
10. |
Piérard GE, Arrese JE, De Doncker P. Antifungal activity of itraconazole and terbinafine in human stratum corneum: A comparative study. J Am Acad Dermatol 1995;32:429-35.
[Google Scholar]
|
11. |
Faergemann J, Laufen H. Levels of fluconazole in serum, stratum corneum, epidermis-dermis (without stratum corneum) and eccrine sweat. Clin Exp Dermatol 1993;18:102-6.
[Google Scholar]
|
12. |
Wildfeuer A, Faergemann J, Laufen H, Pfaff G, Zimmermann T, Seidl HP, et al. Bioavailability of fluconazole in the skin after oral medication. Mycoses 1994;37:127-30.
[Google Scholar]
|
13. |
Hau CS, Tada Y, Kanda N, Watanabe S. Immunoresponses in dermatomycoses. J Dermatol 2015;42:236-44.
[Google Scholar]
|
14. |
Miskeen AK, Uppuluri P. Laboratory diagnosis of fungal infections: A primer. In: Sardana K, Mahajan K, Arora P, editors. Fungal Infections: Diagnosis and Management. Delhi: CBS; 2017. p. 23-39.
[Google Scholar]
|
15. |
Ardeshna KP, Rohatgi S, Jerajani HR. Successful treatment of recurrent dermatophytosis with isotretinoin and itraconazole. Indian J Dermatol Venereol Leprol 2016;82:579-82.
[Google Scholar]
|
16. |
de Souza Leão Kamamoto C, Sanudo A, Hassun KM, Bagatin E. Low-dose oral isotretinoin for moderate to severe seborrhea and seborrheic dermatitis: A randomized comparative trial. Int J Dermatol 2017;56:80-5.
[Google Scholar]
|
Fulltext Views
3,791
PDF downloads
1,712